top of page
RESEARCH
As a physics PhD student at UC Santa Barbara, I split my time between designing cutting edge optics for direct imaging and using existing instruments to characterize exoplanet atmospheres.
As an undergraduate and engineering masters student, I was fortunate enough to study at an Ivy League institution and work on several astrophysics projects. These projects greatly varied in focus, from dark matter and the epoch of reionization to instrumental noise reduction to exoplanet characterization.
Between these projects and my current work, I have developed strong enthusiasm for observational exoplanetology and the technology behind observing systems. Read on to find out more about what I have been working on!
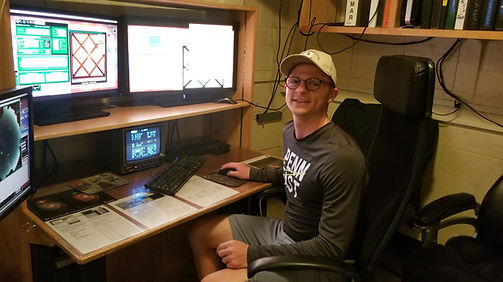
Working with Assistant Prof. Max Millar-Blanchaer at UC Santa Barbara, I have been developing cutting edge optics for implementation in exoplanet direct-imaging systems. These optical devices, known as metasurfaces, offer high precision phase, transmission, and polarization control that can greatly enhance our direct imaging capabilities. And perhaps equally as exciting: to our knowledge, they have never been used for any astronomical application so far!
Metasurfaces are arrays of thousands of nanoscale structures that can reflect or transmit light. The physical properties of a given nanostructure, including size, shape, and material, dictate how that nanostructure can manipulate incident light. When you distribute thousands - or millions - of these structures, each with distinct physical properties, in a particular arrangement, you can create an optic that performs any number of functions at an incredibly high resolution.
Currently, I am designing a metasurface phase-apodizing coronagraph, or a metasurface that can block starlight so that we can see and image planets orbiting other stars. Should we demonstrate the success of such a device, the gate can be opened for countless other astronomical applications. Pictured above is a simulation of light transmitting through the metasurface coronagraph device.
Working with Assistant Prof. Max Millar-Blanchaer at UC Santa Barbara, I am also leading spectroscopic observations of gas-giant planets in an effort to understand their cloud structure, composition, and dynamics.
Characterizing exoplanets is essential to our understanding of our Solar System's place among the billions of other existing star planet systems. At the present, technological limitations prevent detailed atmospheric characterization of terrestrial planets, but some gaseous planets are both large enough and bright enough to reveal details of their subsurface workings to Earth-based observatories. Such details can enhance our understanding of planetary formation as well as contribute to a growing directory of the kinds of atmospheres that planets outside of our Solar System can host.
By measuring the brightness of a given planet over a range of different wavelengths over a span of time, characteristics of the planet's cloud composition can be deduced. Variability in brightness over time can indicate the presence of cloud features that enhance/reduce planetary brightness as they rotate in and out of view (as the planet rotates about its axis). Strong variability at one particular wavelength of light versus another can indicate the chemical composition of planetary clouds, because strong changes in brightness at a wavelength that a particular chemical may like to absorb (or prevent us from seeing) may indicate that clouds composed of that chemical are moving in and out of view over time.
I am currently working on determining these characteristics for the giant (~10 Jupiter mass) planet VHS1256b. Using spectroscopic observations taken with the Keck MOSFIRE instrument, I am in the midst of prcoessing and analyzing data such that it can be used to detect variability and thus atmospheric information. Above is a direct image of the VHS1256 star-planet system taken from this website.
UNDERGRAD PROJECTS
At Columbia University, I have been working with Professor David Kipping on coming up with inventive ways to examine public radial velocity (RV) data with the intention of validating new exoplanets. We have invented our own validation technique using archival data and used it to validate one new planet, HD 183579b! Our research has been accepted for publication in Astrophysical Journal Letters, and is available publicly at this link. The above animation is my depiction of the warm-Neptune type exoplanet. Click on the "Exoplanets" button above, or head to the "science media" page to see a video Prof. Kipping made about our planet!
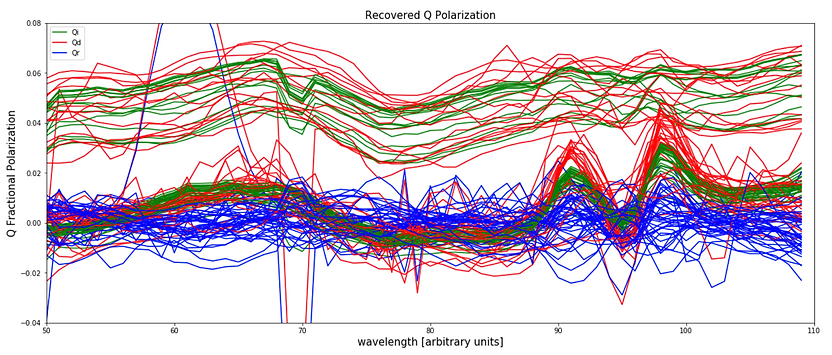
At Caltech, as a Student Undergraduate Research Fellow, I worked with Dr. Dimitri Mawet and Dr. Max Millar-Blanchaer on a spectropolarimetry project. Spectropolarimetry, or the analysis of the spectra of polarized light, is a useful tool for learning about the cloud composition and structure of brown-dwarfs, which are great analogs for gas-giant exoplanets.
The Wide-field InfraRed Camera+Polarimeter (WIRC+Pol) at Palomar Observatory has been collecting spectropolarimetric data on brown-dwarfs and stars since March 2017, but much of the early data was unable to be used for science due to high levels of systematic noise hiding the polarized signals. This noise came from a combination of various factors, including telescope pointing and the position of the target object on the detector.
I reduced these systematics from as high as 8% to below 1% by modeling the noise as a function of various telescope parameters and subtracting this model from the data. The animation above visualizes how the WIRC+Pol instrument takes data, and the figure above shows the polarization signal (in blue) recovered by subtracting the noise model (in green) from the data (in red) for several standard stars.
At Penn, I worked with three other students under Dr. Adam Lidz investigating the effects of dark matter on 21cm wavelength astrophysical radiation by predicting output of the Hydrogen Epoch of Reionization Array (HERA).
HERA is a massive radio telescope array located in South Africa that is aiming to measure the 21cm radiation signal emitted at various cosmological redshifts. 21cm radiation can reveal information about the period in the universe's evolution known as the Epoch of Reionization.
The gravitational effects of different types of dark matter change the way the 21cm signal evolves with redshift, so measuring the 21cm signal can also reveal insights into the nature of dark matter in the universe.
To this effect, we simulated 21cm signals for two different models of dark matter, cold dark matter (CDM) and fuzzy dark matter (FDM). When HERA begins to collect data, our results may be used to help interpret the signals being received, and by doing this potentially allow scientists to gain a better understanding of the structure of dark matter. The video above shows how the 21cm signal evolves with decreasing redshift for the CDM and FDM cases. Our results were accepted for publication by the Astrophysical Journal, and the preprint is available here.
bottom of page